Alamethicin
* Please be kindly noted products are not for therapeutic use. We do not sell to patients.

Category | Antibiotics |
Catalog number | BBF-04141 |
CAS | 27061-78-5 |
Molecular Weight | 1964.30 |
Molecular Formula | C92H150N22O25 |
Online Inquiry
Capabilities & Facilities
Fermentation Lab
4 R&D and scale-up labs
2 Preparative purification labs
Fermentation Plant
Semi pilot, pilot and industrial plant 4 Manufacturing sites 7 Production lines at pilot scale 100+ Reactors of 30-4000 L; 170+ reactors of 20 KL-30 KL; 24+ reactors of >100 KL 2 Hydrogenation reactors (200 L, 4Mpa and 1000L, 4Mpa)
Product Description
Alamethicin, an antibiotic peptide compound, could be obtained from the fungus Trichoderma viride and is commonly used in studing ion channel assembly and peptide-membrane interactions.
- Specification
- Properties
- Reference Reading
- Price Product List
Sequence | XPXAXAQXVXGLXPVXXEQF |
Storage | Store at -20°C (dark) |
IUPAC Name | (4S)-4-[[2-[[2-[[(2S)-2-[[(2S)-1-[2-[[(2S)-2-[[2-[[2-[[(2S)-2-[[2-[[(2S)-2-[[(2S)-2-[[2-[[(2S)-2-[[2-[[(2S)-1-(2-acetamido-2-methylpropanoyl)pyrrolidine-2-carbonyl]amino]-2-methylpropanoyl]amino]propanoyl]amino]-2-methylpropanoyl]amino]propanoyl]amino]-5-amino-5-oxopentanoyl]amino]-2-methylpropanoyl]amino]-3-methylbutanoyl]amino]-2-methylpropanoyl]amino]acetyl]amino]-4-methylpentanoyl]amino]-2-methylpropanoyl]pyrrolidine-2-carbonyl]amino]-3-methylbutanoyl]amino]-2-methylpropanoyl]amino]-2-methylpropanoyl]amino]-5-[[(2S)-5-amino-1-[[(2R)-1-hydroxy-3-phenylpropan-2-yl]amino]-1,5-dioxopentan-2-yl]amino]-5-oxopentanoic acid |
Canonical SMILES | CC(C)CC(C(=O)NC(C)(C)C(=O)N1CCCC1C(=O)NC(C(C)C)C(=O)NC(C)(C)C(=O)NC(C)(C)C(=O)NC(CCC(=O)O)C(=O)NC(CCC(=O)N)C(=O)NC(CC2=CC=CC=C2)CO)NC(=O)CNC(=O)C(C)(C)NC(=O)C(C(C)C)NC(=O)C(C)(C)NC(=O)C(CCC(=O)N)NC(=O)C(C)NC(=O)C(C)(C)NC(=O)C(C)NC(=O)C(C)(C)NC(=O)C3CCCN3C |
InChI | InChI=1S/C92H150N22O25/c1-47(2)43-58(72(127)108-92(24,25)84(139)113-41-29-33-59(113)73(128)103-65(48(3)4)75(130)111-90(20,21)82(137)112-89(18,19)80(135)102-56(37-40-64(120)121)70(125)101-55(35-38-61(93)117)69(124)98-54(46-115)44-53-31-27-26-28-32-53)99-63(119)45-95-77(132)85(10,11)110-76(131)66(49(5)6)104-81(136)88(16,17)107-71(126)57(36-39-62(94)118)100-67(122)50(7)96-78(133)86(12,13)106-68(123)51(8)97-79(134)87(14,15)109-74(129)60-34-30-42-114(60)83(138)91(22,23)105-52(9)116/h26-28,31-32,47-51,54-60,65-66,115H,29-30,33-46H2,1-25H3,(H2,93,117)(H2,94,118)(H,95,132)(H,96,133)(H,97,134)(H,98,124)(H,99,119)(H,100,122)(H,101,125)(H,102,135)(H,103,128)(H,104,136)(H,105,116)(H,106,123)(H,107,126)(H,108,127)(H,109,129)(H,110,131)(H,111,130)(H,112,137)(H,120,121)/t50-,51-,54+,55-,56-,57-,58-,59-,60-,65-,66-/m0/s1 |
InChI Key | LGHSQOCGTJHDIL-UTXLBGCNSA-N |
Source | Trichoderma viride |
Appearance | Off-white to Beige Powder |
Application | Can mimic nerve action potential across artificial membranes. |
Boiling Point | 2090.0±65.0°C at 760 mmHg |
Melting Point | 252-272°C |
Density | 1.2±0.1 g/cm3 |
Solubility | Soluble in DMSO, Methanol, Ethanol |
BBF-03753 | Baicalin | Inquiry |
BBF-02582 | Polyporenic acid C | Inquiry |
BBF-03428 | Tubermycin B | Inquiry |
BBF-00969 | Hygromycin A | Inquiry |
BBF-01825 | Loganin | Inquiry |
BBF-05880 | N-Me-L-Ala-maytansinol | Inquiry |
Bio Calculators
* Our calculator is based on the following equation:
Concentration (start) x Volume (start) = Concentration (final) x Volume (final)
It is commonly abbreviated as: C1V1 = C2V2
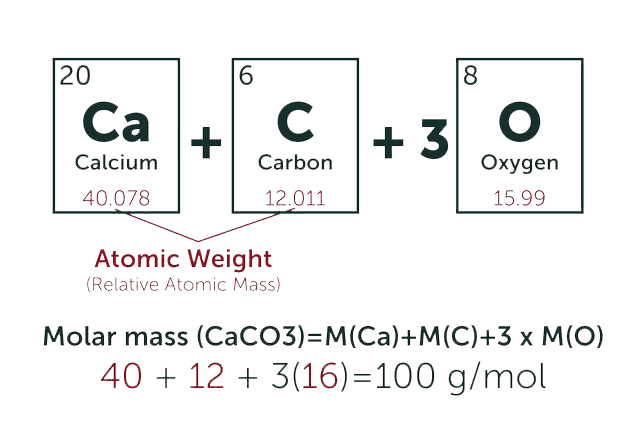