Manumycin
* Please be kindly noted products are not for therapeutic use. We do not sell to patients.

Category | Antibiotics |
Catalog number | BBF-02660 |
CAS | 52665-74-4 |
Molecular Weight | 550.64 |
Molecular Formula | C31H38N2O7 |
Purity | ≥98% |
Online Inquiry
Description
It is produced by the strain of Str. parvullus ETH 25000. It has anti-gram-positive bacterial effect, and has weak antibacterial effect on some mycobacteria and candida.
Specification
Synonyms | Manumycin A; NSC 622141; Antibiotic UCF 1C; 2,4-Decadienamide, N-[(1S,5S,6R)-5-hydroxy-5-[(1E,3E,5E)-7-[(2-hydroxy-5-oxo-1-cyclopenten-1-yl)amino]-7-oxo-1,3,5-heptatrienyl]-2-oxo-7-oxabicyclo[4.1.0]hept-3-en-3-yl]-2,4,6-trimethyl-, (2E,4E,6R)-; (-)-Manumycin A; Antibiotic TMC 1F |
Storage | Store at 2-8°C |
IUPAC Name | (2E,4E,6R)-N-[(1S,5S,6R)-5-hydroxy-5-[(1E,3E,5E)-7-[(2-hydroxy-5-oxocyclopenten-1-yl)amino]-7-oxohepta-1,3,5-trienyl]-2-oxo-7-oxabicyclo[4.1.0]hept-3-en-3-yl]-2,4,6-trimethyldeca-2,4-dienamide |
Canonical SMILES | CCCCC(C)C=C(C)C=C(C)C(=O)NC1=CC(C2C(C1=O)O2)(C=CC=CC=CC(=O)NC3=C(CCC3=O)O)O |
InChI | InChI=1S/C31H38N2O7/c1-5-6-11-19(2)16-20(3)17-21(4)30(38)32-22-18-31(39,29-28(40-29)27(22)37)15-10-8-7-9-12-25(36)33-26-23(34)13-14-24(26)35/h7-10,12,15-19,28-29,34,39H,5-6,11,13-14H2,1-4H3,(H,32,38)(H,33,36)/b8-7+,12-9+,15-10+,20-16+,21-17+/t19-,28-,29-,31+/m1/s1 |
InChI Key | TWWQHCKLTXDWBD-MVTGTTCWSA-N |
Properties
Appearance | Pale Yellow Crystal |
Antibiotic Activity Spectrum | Gram-positive bacteria; Mycobacteria; Yeast |
Boiling Point | 863.6±65.0°C (Predicted) |
Melting Point | 139-141°C (dec.) |
Density | 1.26±0.1 g/cm3 (Predicted) |
Solubility | Soluble in Chloroform, Ethanol, Methanol, Acetonitrile, Benzene |
Reference Reading
1. Manumycin enhances the cytotoxic effect of paclitaxel on anaplastic thyroid carcinoma cells
A Bamiagis, J Pan, M Christgen, G Xu, S C Yeung Cancer Res . 2000 Feb 1;60(3):650-6.
Despite the current multimodal approach to treatment of anaplastic thyroid cancer (ATC), the prognosis for patients with the disease is poor. New effective therapy for ATC is desperately needed. Thus, we investigated the effects of manumycin (a farnesyl:protein transferase inhibitor), alone and in combination with other drugs frequently used to treat ATC, in six human ATC cell lines: ARO, C643, DRO, Hth-74, KAT-4, and KAT-18. By means of a formazan dye-based spectrophotometric assay of cell viability and light microscopy, manumycin was shown to decrease the number of viable cells in all six of the cell lines though to a lesser degree in DRO and C643 cells than in ARO, Hth-74, KAT-4, and KAT-18 cells. In combination, manumycin enhanced the effect of paclitaxel in all six of the cell lines. The mechanism of cell death was investigated by measuring caspase-3 activity, immunoblotting with anti-poly-(ADP-ribose)polymerase (PARP) antibody and electrophoresis of DNA. After an 18-h incubation, manumycin plus paclitaxel caused enhanced activation of caspase-3 activity, cleavage of PARP into Mr 89,000 and 28,000 fragments, and internucleosomal fragmentation of DNA (all of which are characteristic of apoptotic cell death). In contrast, neither manumycin alone, paclitaxel alone, doxorubicin alone, nor doxorubicin plus manumycin produced significant specific cleavage of PARP and internucleosomal DNA fragmentation after 18 h of incubation. The in vivo effect and toxicity of combined manumycin and paclitaxel treatments were evaluated in a nude mouse xenograft model using ARO and KAT-4 cells. Drugs were injected i.p. on days 1 and 3 of a 7-day cycle for three cycles. Both manumycin (7.5 mg/kg/dose) and paclitaxel (20 mg/kg/dose) had significant inhibitory effects on tumor growth. Combined manumycin and paclitaxel treatments seemed as effective as manumycin against ARO cells and more effective than either manumycin or paclitaxel alone against KAT-4 cells. No significant morbidity or mortality was caused by the treatments. In conclusion, manumycin can inhibit the growth of ATC both in vitro and in vivo. Manumycin plus paclitaxel has enhanced cytotoxic effects and increased apoptotic cell death in ATC cells in vitro compared with either drug by itself. The combination of manumycin and paclitaxel is also effective in vivo with no significant toxicity observed. The lack of synergy observed in this in vivo experiment may be due to a ceiling effect, and further experimentation is warranted to ascertain the optimal way to combine these two agents for maximal therapeutic effects.
2. Isolation of manumycin-type derivatives and genome characterization of a marine Streptomyces sp. C1-2
Chakapong Intaraudom, Pattama Pittayakhajonwut, Duangkamol Ruen-Ngam, Jaturong Pratuangdejkul, Chitti Thawai, Sakkarn Chanadech, Sumet Chongruchiroj Res Microbiol . 2021 Mar;172(2):103812. doi: 10.1016/j.resmic.2021.103812.
A marine actinomycete strain C1-2 was taxonomically characterized as the genus Streptomyces, based on whole-genome sequence analysis. The highest average nucleotide identity (ANI) value (98.96%) and digital DNA-DNA hybridization (DDH) value (90.4%) were observed between Streptomyces sp. C1-2 and Streptomyces griseoaurantiacus. Thus, Streptomyces sp. C1-2 could be identified as S. griseoaurantiacus. Genome analysis revealed that Streptomyces sp. C1-2 contained 22 biosynthetic gene clusters (BGCs) for secondary metabolites, where among them, 54% have low similarities with known BGCs. The chemical investigation led to the isolation of three new manumycin-type derivatives and two known analog antibiotics named SW-B and cornifronin B. All compounds showed antioxidant activity with the half-maximal inhibitory concentration (IC50) values in a range of 50.82 ± 0.8-112.04 ± 1.0 μg/mL with no cytotoxicity against Vero cells. This is the first report of the antioxidant property of manumycin-type derivatives. Moreover, two known compounds exhibited antifungal activity against Phytophthora capsici, Fusarium oxysporum f. sp. cucumerinum, and Magnaporthe grisea, with the minimum inhibitory concentration (MIC) values in a range of 125-500 μg/mL.
3. Inhibiting extracellular vesicles formation and release: a review of EV inhibitors
Lorraine O'Driscoll, Mariadelva Catalano J Extracell Vesicles . 2019 Dec 19;9(1):1703244. doi: 10.1080/20013078.2019.1703244.
It is now becoming well established that vesicles are released from a broad range of cell types and are involved in cell-to-cell communication, both in physiological and pathological conditions. Once outside the cell, these vesicles are termed extracellular vesicles (EVs). The cellular origin (cell type), subcellular origin (through the endosomal pathway or pinched from the cell membrane) and content (what proteins, glycoproteins, lipids, nucleic acids, metabolites) are transported by the EVs, and their size, all seem to be contributing factors to their overall heterogeneity. Efforts are being invested into attempting to block the release of subpopulations of EVs or, indeed, all EVs. Some such studies are focussed on investigating EV inhibitors as research tools; others are interested in the longerterm potential of using such inhibitors in pathological conditions such as cancer. This review, intended to be of relevance to both researchers already well established in the EV field and newcomers to this field, provides an outline of the compounds that have been most extensively studied for this purpose, their proposed mechanisms of actions and the findings of these studies.
Recommended Products
BBF-01825 | Loganin | Inquiry |
BBF-04609 | 1,1-Dimethylbiguanide hydrochloride | Inquiry |
BBF-02800 | DB-2073 | Inquiry |
BBF-00968 | Homoalanosine | Inquiry |
BBF-03428 | Tubermycin B | Inquiry |
BBF-03488 | Streptozotocin | Inquiry |
Bio Calculators
* Our calculator is based on the following equation:
Concentration (start) x Volume (start) = Concentration (final) x Volume (final)
It is commonly abbreviated as: C1V1 = C2V2
* Total Molecular Weight:
g/mol
Tip: Chemical formula is case sensitive. C22H30N4O √ c22h30n40 ╳
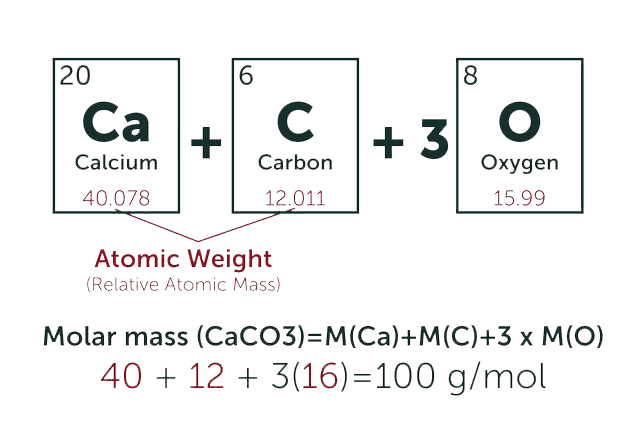